The concept that “the key to the future lies in the past” resonates across almost all disciplines but holds especially true for the climate sciences. Since high-quality, instrumental climate records only date back ~150 years, we have to rely on the geologic record to understand how climate has naturally changed in the past. By reconstructing past climate conditions, we can understand how the Earth system responds to increased sea surface temperatures, zero ice cover, and most important for constraining future climate change: elevated atmospheric carbon dioxide (CO2) concentrations (Figure 1). However, obtaining climate information from the geologic record is not trivial, and direct, quantitative records of past climate are rare.
Therefore, we use paleoclimate proxies (i.e., surrogates for climate variables that cannot be directly measured; Tierney et al., 2021) to produce qualitative and quantitative records of past climate. There are four components to a paleoclimate proxy (Evans et al., 2013):
Environment: The environmental parameter that affects the sensor.
Sensor: The physical, chemical, and/or biological components that respond to environmental changes.
Archive: The medium in which the response of a sensor to an environmental change is recorded.
Observation: What we measure from archives, and what we generally refer to as the proxy. We use observations to estimate the environmental response that the sensor imprinted on the archive.
For example, changes in global air temperatures (environment) influence the size and extent of ice sheets (sensor). We can collect ice cores (archive) and use the stable isotopic composition (observation) of air trapped in the core as a paleotemperature proxy (Severinghaus & Brook, 1999). Another creative example is that the carbon isotopic composition (δ13C) of bat guano (i.e., accumulated excrement) has been used as a proxy for paleoclimate and paleovegetation cover (Onac et al., 2014). As new proxy systems continue to be discovered/developed, our understanding of the mechanisms modulating Earth’s climate system becomes more and more refined. It is important to note that paleoclimate proxy systems filter natural climate signals differently, so multiple proxy types are often utilized when reconstructing regional and global climate. Yet, the question remains: how do we use information about past climates to understand recent and future climate change? To answer this question, I will use my current research as an example.
There is the lack of continuous paleoclimate and paleovegetation records that come from South America, especially the Amazon Basin (a region where environmental changes have implications for the global carbon and hydrologic cycles; Gatti et al., 2021; Davidson et al., 2012), relative to North America and Europe (Figure 2). Consequently, we lack long-term baseline data on natural climate variability in Amazonia and its connections with other regional and global climate systems—highlighting a critical need to generate new paleoclimate records in Amazonia and to investigate landscape impacts of past climate changes. My research uses organic biomarkers (i.e., compounds/compound classes preserved in geological materials that can be traced to a particular organism, group of organisms, or a specific process; Castañeda & Shouten, 2013) preserved in lake sediments to reconstruct South American hydroclimate and environmental conditions, and to understand why they changed. Lake sediments are composed of clastic materials, plant/organic matter, and other mobile compounds that are transported via water and wind from the landscape to lakes. These sediments continuously accumulate at the bottom of lakes forming layers, meaning that the deeper you go into the sediment, the further you go back in time. Absolute dating techniques (e.g., radiocarbon and lead-210) of the sediments are then performed to understand when deposition occurred (Figure 3).
My current project uses the hydrogen isotopic composition (δ2H) of plant-wax biomarkers (e.g., n-alkanes) preserved in lake sediments from Lago Caranã, a small lake in the heart of the Brazilian Amazon Rainforest, to reconstruct Amazonian hydroclimate (paleoprecipitation) over the past ~5,600 years. Additionally, humans have been present in Amazonia for over 13,000 years (Roosevelt, 2013). They have altered regional forest composition and structure via agroforestry practices and controlled burnings (Maezumi et al., 2018). The lasting impacts of human-induced landscape changes in the region can complicate paleoclimate interpretations, as changes in climate influence vegetation cover. Therefore, my research will reconstruct vegetation cover at Lago Caranã using the δ13C of plant-wax biomarkers and existing pollen data (Maezumi et al., 2018). Fire biomarkers (e.g., PAHs and levoglucosan, charcoal counts, and human fecal sterols from Lago Caranã sediments) can then pinpoint when humans were present in the region, providing the potential to disentangle human and climate-driven ecological changes.
To answer the question mentioned above, the results from this study can be used to evaluate climate model estimates of past hydroclimate variability, which will improve our understanding of the processes driving future South American climate change. Information about the past range and rates of climate change are captured in paleoclimate and paleoenvironmental records. Thus, using these records to make quantitative, accurate interpretations of climate change on geologic timescales, extending beyond the reach of the narrow historical record, can improve our understanding of natural climate mechanisms and how they respond to anthropogenic forcing. In the context of Amazonia, understanding how future climate change will influence precipitation and vegetation dynamics is critical, as projected drying in Amazonia would have devastating effects on the communities and ecosystems that depend on freshwater resources linked to precipitation seasonality, including the Sateré-Mawé, Munduruku, and Mebêngôkre indigenous societies.

Figure 1: Figure and caption from Tierney et al. (2021). Paleoclimate context for future climate scenarios. Global mean surface temperature for the past 100 million years is estimated from benthic δ18O using the method of Hansen et al. (2013). CO2 is estimated from the multiproxy dataset compiled by Foster et al. (2017), with phytane data from Witkowski et al. (2018) and boron data from Sosdian et al. (2018) and Henehan et al. (2019). Data with unrealistic values (<150 ppm) are excluded. The CO2 error envelopes represent 1σ uncertainties. Note the logarithmic scale for CO2. Gaussian smoothing was applied to both the temperature and CO2 curves to emphasize long-term trends. Temperature colors are scaled relative to preindustrial conditions. The maps show simplified representations of surface temperature. Projected CO2 concentrations are from the extended SSP scenarios (Meinshausen et al., 2019). Blue bars indicate when there are well-developed ice sheets (solid lines) and intermittent ice sheets (dashed lines), according to previous syntheses (Zachos et al., 2001). NH, Northern Hemisphere.


Figure 2: From Gross & Wahl. (2017): Geographic coverage of World Data Service for paleoclimate data
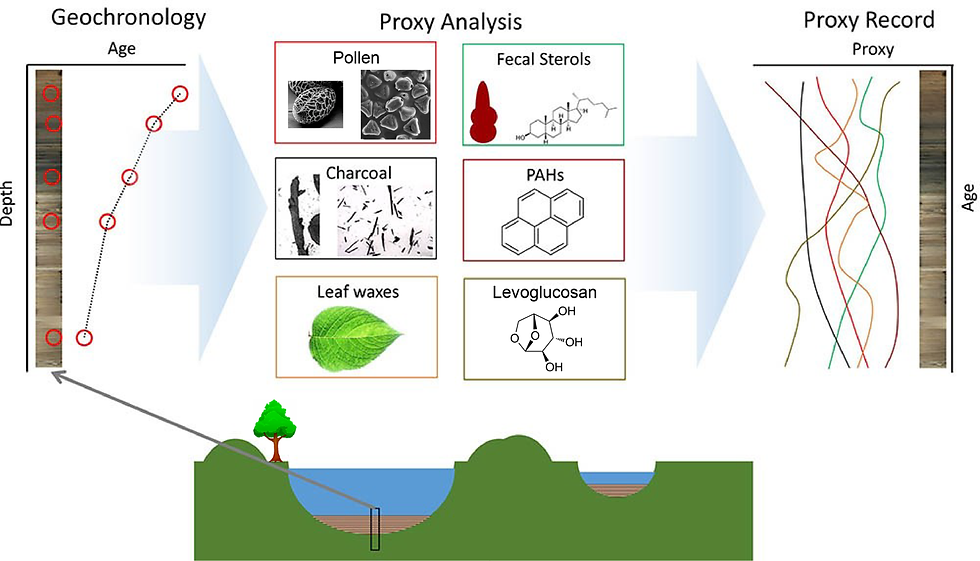
Figure 3: Adapted from Vachula et al. 2021. Summary of paleoenvironmental analysis of lake sediment records. First, the age-depth relationships of sediment cores are established using geochronological techniques. Next, proxies in the sediment, such as pollen, fecal sterols, charcoal, polycyclic aromatic hydrocarbons, leaf waxes, or levoglucosan, are analyzed to develop proxy records. These proxy records are then used to infer how climatic and/or environmental conditions have changed through time.
Works Cited (MLA 8th Edition):
Castañeda, Isla S., and Stefan Schouten. “A Review of Molecular Organic Proxies for Examining Modern and Ancient Lacustrine Environments.” Quaternary Science Reviews, vol. 30, no. 21–22, Oct. 2011, pp. 2851–91. DOI.org (Crossref), https://doi.org/10.1016/j.quascirev.2011.07.009.
Davidson, Eric A., et al. “The Amazon Basin in Transition.” Nature, vol. 481, no. 7381, Jan. 2012, pp. 321–28. DOI.org (Crossref), https://doi.org/10.1038/nature10717.
Evans, M. N., et al. “Applications of Proxy System Modeling in High Resolution Paleoclimatology.” Quaternary Science Reviews, vol. 76, Sept. 2013, pp. 16–28. DOI.org (Crossref), https://doi.org/10.1016/j.quascirev.2013.05.024.
Foster, Gavin L., et al. “Future Climate Forcing Potentially without Precedent in the Last 420 Million Years.” Nature Communications, vol. 8, no. 1, Apr. 2017, p. 14845. www.nature.com, https://doi.org/10.1038/ncomms14845.
Gatti, Luciana V., et al. “Amazonia as a Carbon Source Linked to Deforestation and Climate Change.” Nature, vol. 595, no. 7867, July 2021, pp. 388–93. www.nature.com, https://doi.org/10.1038/s41586-021-03629-6.
Gross, Wendy, and Eugene Wahl. “Finding Paleoclimate Data via the World Data Service for Paleoclimatology Just Got Easier.” World Data System Blog, https://www.worlddatasystem.org/news/blog/finding-paleoclimate-data-via-wds-paleoclimatology-just-got-easier. Accessed 2 Nov. 2021.
Hansen, James, et al. “Climate Sensitivity, Sea Level and Atmospheric Carbon Dioxide.” Philosophical Transactions of the Royal Society A: Mathematical, Physical and Engineering Sciences, vol. 371, no. 2001, Oct. 2013, p. 20120294. DOI.org (Crossref), https://doi.org/10.1098/rsta.2012.0294.
Henehan, Michael J., et al. “Rapid Ocean Acidification and Protracted Earth System Recovery Followed the End-Cretaceous Chicxulub Impact.” Proceedings of the National Academy of Sciences, vol. 116, no. 45, Nov. 2019, pp. 22500–04. DOI.org (Crossref), https://doi.org/10.1073/pnas.1905989116.
Maezumi, S. Yoshi, et al. “New Insights From Pre-Columbian Land Use and Fire Management in Amazonian Dark Earth Forests.” Frontiers in Ecology and Evolution, vol. 6, Aug. 2018, p. 111. DOI.org (Crossref), https://doi.org/10.3389/fevo.2018.00111.
Meinshausen, Malte, et al. “The Shared Socio-Economic Pathway (SSP) Greenhouse Gas Concentrations and Their Extensions to 2500.” Geoscientific Model Development, vol. 13, no. 8, Aug. 2020, pp. 3571–605. DOI.org (Crossref), https://doi.org/10.5194/gmd-13-3571-2020.
Onac, Bogdan P., et al. “Guano-Derived Δ13C-Based Paleo-Hydroclimate Record from Gaura Cu Musca Cave, SW Romania.” Environmental Earth Sciences, vol. 71, no. 9, May 2014, pp. 4061–69. Springer Link, https://doi.org/10.1007/s12665-013-2789-x.
Roosevelt, A. C. “The Amazon and the Anthropocene: 13,000 Years of Human Influence in a Tropical Rainforest.” Anthropocene, vol. 4, Dec. 2013, pp. 69–87. DOI.org (Crossref), https://doi.org/10.1016/j.ancene.2014.05.001.
Severinghaus, Jeffrey P., and Edward J. Brook. “Abrupt Climate Change at the End of the Last Glacial Period Inferred from Trapped Air in Polar Ice.” Science, vol. 286, no. 5441, Oct. 1999, pp. 930–34. DOI.org (Crossref), https://doi.org/10.1126/science.286.5441.930.
Sosdian, S. M., et al. “Constraining the Evolution of Neogene Ocean Carbonate Chemistry Using the Boron Isotope PH Proxy.” Earth and Planetary Science Letters, vol. 498, Sept. 2018, pp. 362–76. DOI.org (Crossref), https://doi.org/10.1016/j.epsl.2018.06.017.
Tierney, Jessica E., et al. “Past Climates Inform Our Future.” Science, vol. 370, no. 6517, Nov. 2020, p. eaay3701. DOI.org (Crossref), https://doi.org/10.1126/science.aay3701.
Vachula, Richard, et al. “New Approaches to Study Interactions Among Climate, Environment, and Humans in Arctic Alaska.” Alaska Park Science, vol. 20, no. 1, 2021, pp. 2–9.
Witkowski, Caitlyn R., et al. “Molecular Fossils from Phytoplankton Reveal Secular P co 2 Trend over the Phanerozoic.” Science Advances, vol. 4, no. 11, Nov. 2018, p. eaat4556. DOI.org (Crossref), https://doi.org/10.1126/sciadv.aat4556.
Zachos, James, et al. “Trends, Rhythms, and Aberrations in Global Climate 65 Ma to Present.” Science, vol. 292, no. 5517, Apr. 2001, pp. 686–93. DOI.org (Crossref), https://doi.org/10.1126/science.1059412.
Kommentare